Research
Materials Informatics
Transition Pathway sampling via swarm intelligence and graph theory
Despite the progress on the prediction of (meta)stable materials, the lack of the capability to predict the synthesizability remains the biggest challenge in the computational materials design. According to transition state theory, the thermodynamic properties of transition states can be used to quantify chemical reaction rates and to identify possible synthetic pathways, which was considered to be extremely challenging. To overcome this obstacle, I developed a new pathway sampling approach via swarm-intelligence and graph theory (PALLAS method), which establishes low-energy transition pathways between minima without specification of any mechanistic details a priori. This technique integrates the solid-state dimer method, a robust fingerprint method that can accurately quantify the dissimilarity between crystal structures, structure searching through the swarm-intelligence algorithm, and graph theory to automatically analyze transition paths, and find the best solution. The capabilities of the PALLAS method are promising as a major tool for materials by design and can provide many metastable structures with kinetic information, which is useful for screening whether new phases can be synthesized. Figure 1 shows an actual result predicted by our PALLAS method for silicon.
[J. Phys. Chem. Lett. 10, 5019 (2019)]
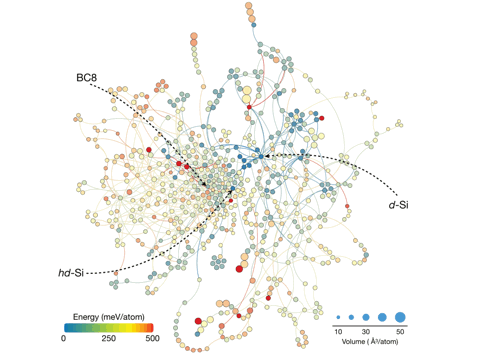
Fingerprint based metric for comparing materials across structural space
The structure predictions can produce a huge number of new structures that have either not yet been found experimentally or are metastable. A structural similarity metric is crucial for classifying structures, searching chemical space for better compounds and materials, and driving the next generation of machine-learning techniques. As a lead developer, I developed a reliable fingerprint method for measuring similarities of crystalline structures, providing powerful metrics that enable the navigation of structural space within a unified framework. This fingerprint method can also be used to accurately explore local environments for machine learning techniques, which is expected to find major applications in materials discovery.
[J. Chem. Phys. 144, 034203 (2016)]
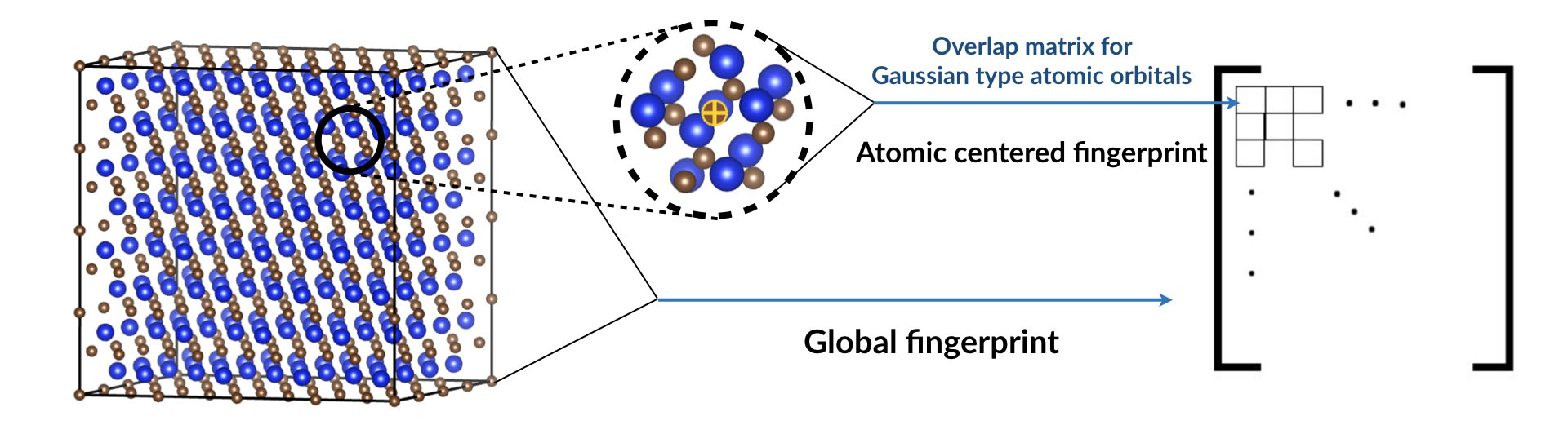
Computational Materials Design
The discovery and implementation of novel materials play an essential role in the development of modern technology. New materials have historically been discovered by either trial-and-error processes or serendipity, both of which are a painstakingly long and costly process. With recent advances in computational tools, we are now able to predict new materials and their physical properties with remarkable accuracy. Structural design of novel functional materials through structure prediction and first-principles calculations is one of my main research directions for accelerating materials discovery.
Carbon-boron clathrates as a new class of sp3-bonded framework materials. Carbon-based frameworks comprised of sp3 bonding represent a class of extremely lightweight strong materials, but only diamond and a handful of other compounds exist despite numerous predictions. Using our swarm-intelligence-based structure searching algorithm, we predict the first thermodynamically stable carbon-based clathrate in the bipartite sodalite structure (type VII clathrate), SrB3C3, which is synthesized subsequently. The realization of a carbon-based clathrate creates opportunities for new materials with excellent physical properties, and the stabilization principle based on boron substitution offers valuable insights into design guidelines for novel carbon-based materials.
[Science Advances 6, eaay8361 (2020)]
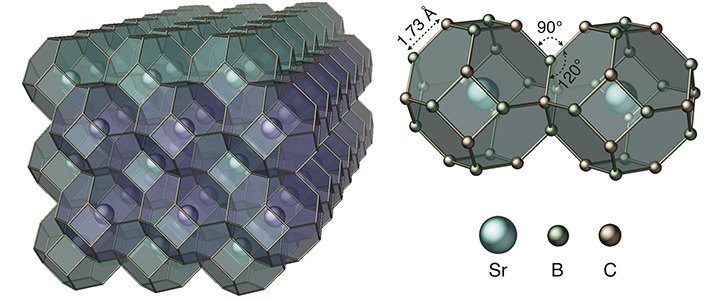
Matter at Extreme States
Pressure is one of the fundamental thermodynamic variables, which can effectively change the interatomic distances and the electrons overlap between two adjacent atoms. As a result, the crystal and electronic structures will be changed forming a series of new high pressure phases with novel physical and chemical properties. Moreover, high pressure can also lower the kinetic energy thus promoting the formation of many new compounds that cannot be formed at normal conditions. High-pressure structure investigation based on structure searching technique in conjunction with first-principles calculations is one of my major research interests.
Reactions of xenon with iron and nickel are predicted in the Earth's inner core.
Studies of the Earth’s atmosphere have shown that more than 90% of xenon (Xe) is depleted compared with its abundance in chondritic meteorites. This long-standing missing Xe paradox has become the subject of considerable interest and several models for a Xe reservoir have been proposed. Whether the missing Xe is hiding in the Earth’s inner core has remained as a long unanswered question. The key to address this issue lies in the reactivity of Xe with iron and nickel (Fe and Ni, the main constituents of the Earth’s core), which has been denied by earlier studies. Here we report on the first prediction of the chemical reaction of Xe with Fe and Ni at the conditions of the Earth’s core through first-principles calculations and unbiased structure searching techniques. We find that Xe and Fe/Ni can form inter-metallic compounds of XeFe3/XeNi3 energetically most stable at the conditions of the Earth’s core. As the result of a Xe → Fe/Ni charge transfer, Xe loses its chemical inertness by opening up the filled 5p electron shell and functioning as a 5p-like element, whilst Fe/Ni is unusually negatively charged, acting as an oxidant rather than a reductant. Our work establishes that the Earth’s inner core is a natural reservoir for Xe storage, and possibly provides the key to unlocking the missing Xe paradox.
[Nature Chem. 6, 644 (2014)]
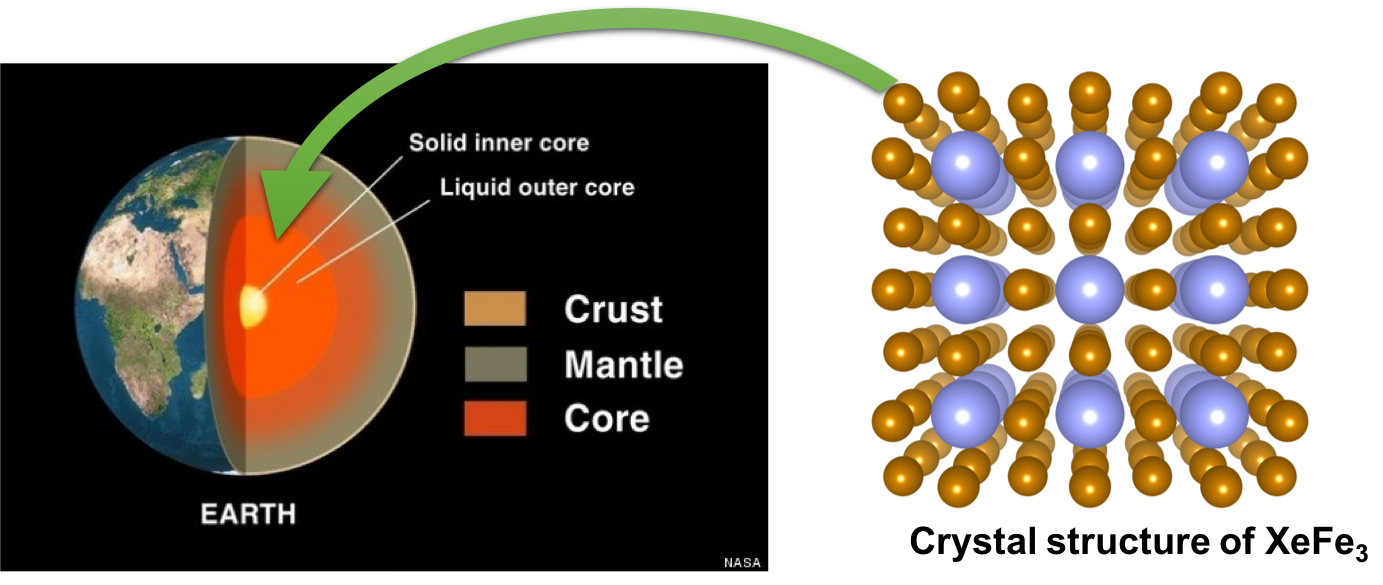